Explaining surround suppression with local competition
Building an anatomically-realistic model for modulation from the visual surround
1st November, 20161112255
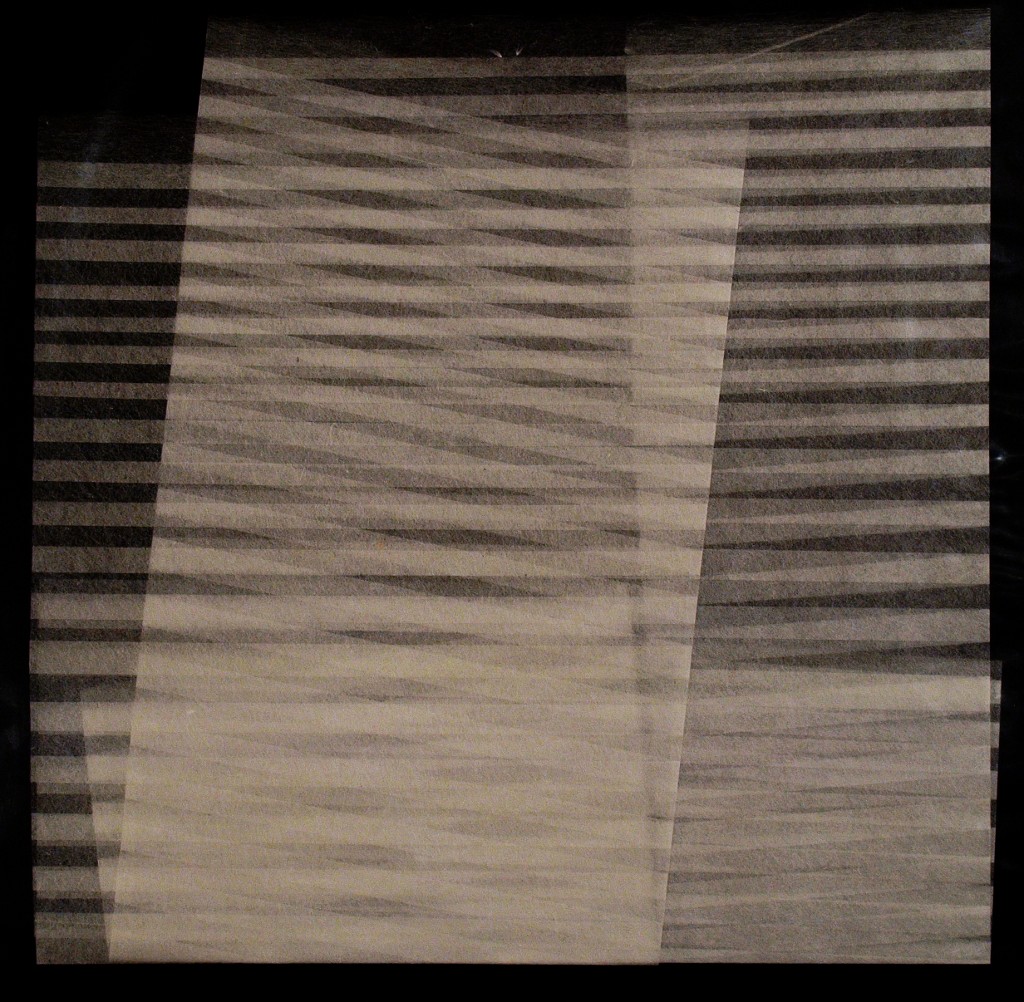
1112255
Existing models for surround suppression in primary visual cortex rely on anatomically-unrealistic inhibitory mechanisms — they incorrectly assume that long-range excitatory projections mainly target inhibitory neurons. We instead hypothesised that local competition, acting within a cortical column, could be recruited by realistic long-range excitatory-to-excitatory connections.
In species with highly developed neocortices, such as cats and primates, cortical neurons are grouped into columns that share functional similarities. In primary visual cortex, columns of neurons have highly similar preferred orientations of visual stimuli. However, given that neurons in a column share the same retinotopic location and have common orientation preferences, their firing activity is surprisingly poorly correlated, even in response to drifting grating stimuli.
We propose that local excitatory neurons group themselves according to higher-order visual properties (e.g. phase, spatial frequency, etc.), and make specific excitatory connections within a column (Fig. 1a). Coupled with local inhibitory feedback, this is a good recipe for setting up competition within a column. We built a model of visual responses in columnar cortex based on this principle (Fig. 1b).
Figure 1: The network architecture of the center-surround model. (a) Connections from single neurons were made both within a column, and between populations. Excitatory neurons within a column were distributed evenly across several local subnetworks (SSNs). A proportion of local excitatory synapses was reserved to be made only with other neurons within the same SSN (\(P_+\)). Long-range excitatory connections were also sensitive to SSN membership, under the parameter \(P_M\). \(J_E, J_I\): Strength of excitatory (\(E\)) and inhibitory (\(I\)) synapses. \(P_{IN}, P_I\): Fraction of synapses onto excitatory (\(IN\)) and inhibitory (\(I\)) targets made locally within the same hypercolumn, as opposed to long-range projections to other hypercolumns. For simplicity, only connections from a single excitatory and inhibitory neuron are shown; projection rules are identical for all neurons in the model. (b) Excitatory (triangles) and inhibitory neurons (circles) in each population were arranged in ordered orientation columns around a ring. Local excitatory and inhibitory connections within each population, as well as long-range excitatory connections between populations, were tuned to preferred orientation (curves in b). For simplicity only projections from a single population are shown (“C” ring; upper); connections were made identically within and between each population in the model. “C”: central visual population; “S”: surround visual population.
Responses to orientation-tuned input in this model (Fig. 2) exhibited identical orientation preferences within columns, as expected. However, temporal correlations between excitatory neurons in different subnetworks were negative, reflecting competition between subnetworks. In addition, stimulating the surround population and the central population simultaneously (Fig. 2b, d) resulted in stronger competition between subnetworks. This was mediated by long-range excitatory projections, which targetted both excitatory and inhibitory neurons.

Figure 2: Neurons within a column are temporally anticorrelated in response to centre-surround grating stimulation. (a–b) Responses of neurons in the centre population, in response to centre-only (a) and centre-surround stimulation (b) with 90° orientation stimulus. Inhibitory neurons: blue-green; two of four excitatory SSNs: red and yellow. (c–d) Firing rates over time for neurons with 90° orientation preference (colours as in a–b). Surround stimulation provokes anticorrelation between subnetworks.
In visual cortex, the strength of suppression induced by wide-field grating stimulation depends on the relative orientations of the grating stimuli presented in the cRF and in the visual surround (Walker et al. 1999). We examined the tuning of suppression in our model by simulating the presentation of two gratings to the centre and surround populations, while varying the relative stimulus orientations (Fig. 3). Consistent with experimental findings, the strongest suppression occurred in our model when the orientations of the center and surround stimuli were aligned (\(\Delta\theta=0\); Fig. 3).
Figure 3: Surround suppression is orientation-tuned, as observed experimentally. Centre-surround grating stimulation (black line: mean response; shading: std. dev.) provokes suppression on average, compared with centre-only stimulation (dashed line: response for centre-only stimulation at preferred orientation). In agreement with experimental findings, our model exhibits orientation-tuned suppression that is strongest when centre and surround orientations are aligned (Walker et al. 1999).
Discussion
The network model we proposed is consistent with the known architecture of columnar cortex, which is characterised by long-range, functionally specific (i.e. orientation specific) but not class-specific lateral excitatory projections, coupled with short-range local inhibition (Kisvárday et al. 1986; McGuire et al. 1991; Kisvárday & Eysel 1993; Binzegger et al. 2004). We include excitatory specificity over a set of local excitatory subnetworks (SSN-specificity) in order to explore the effect of local competition within a cortical column (Muir & Cook 2014; Muir & Mrsic-Flogel 2015).
In our model, suppression provoked by surround stimulation arises through a strictly local competitive mechanism, mediated by strong inhibition within the cortical column. Competition is recruited by subnetwork- and orientation-specific long-range excitatory to excitatory (E\(\rightarrow\)E) projections. As a result, the strongest competition — and therefore the strongest suppression — occurs when the center and surround visual fields are stimulated with gratings of the same orientation. Importantly, long-range excitatory projections in our model are not class-specific; they target excitatory and inhibitory neurons according to their proportions in the cortex.
The mechanism of local competition, recruited by long-rage excitation, explains many features of surround visual stimulation in columnar visual cortex. Local excitatory subnetwork-specificity, over and above orientation-specific connectivity, has not been sought experimentally in mammals with columnar cortices, although it is is important for shaping visual responses in rodent cortex (Cossell et al. 2015). Our results suggest that careful experimental quantification of local circuitry, in functionally-identified neurons, will be important to identify the mechanisms of surround integration in columnar visual cortex.
Publications
Pre-print with methods and more details available from arXiv:1611.00945 [q-bio.NC]. Submission in process.
Funding
This work was supported by the Fundamental Research Funds for the Central Universities (to HY); NSFC (grant 31500863 to HY); the 973 project (grant 2013CB329401 to HY); the European Research council (“Neuromorphic Processors” neuroP project, grant 257219 to GI); and the CSN (fellowships to DRM).
Image credit
111225 by Goran Konjevod. (cc-by-nc)